Industry 4.0 represents the fourth industrial revolution characterised by the integration of digital technologies, the Internet of Things (IoT), artificial intelligence, and advanced robotics into the manufacturing process. This paradigm shift is leading to unprecedented levels of eciency, customization and innovation.
Additive Manufacturing:
At the heart of Industry 4.0 lies Additive Manufacturing, often referred to as 3D printing. This revolutionary technology is redefining how products are designed, prototyped and produced. Unlike traditional subtractive manufacturing processes, additive manufacturing builds objects layer by layer, offering unparalleled design freedom and resource efficiency.
Understanding the Potential of Additive Manufacturing: A Deep Dive
Additive Manufacturing can be traced back to the 1950s when early experiments with layer-by-layer fabrication techniques began.
In the 1970s, researchers like Dr. Hideo Kodama in Japan and Dr. Joseph Beaman in the United States laid the conceptual groundwork for what would later become 3D printing.
The term “3D printing” was coined in the 1980s, and the first patent for stereolithography (SLA) was filed by Chuck Hull in 1984. Hull went on to found 3D Systems Corporation
Around the same time, Carl Deckard and Joe Beaman developed Selective Laser Sintering (SLS) technology, adding to the growing capabilities of Additive Manufacturing.
The 1990s saw the commercialisation of 3D printing technologies, with companies like 3D Systems making SLA machines available to a broader market.
Fused Deposition Modeling (FDM), a widely used 3D printing technology today, was patented by Scott Crump in 1989, and Stratasys, the company founded by Crump, began commercialising FDM in the 1990s.
Now in 2024, 3D bioprinting plays a crucial role in tissue engineering and has the potential to address numerous problems in areas such as healthcare and life sciences. It aims to fabricate functional tissue for applications in regenerative medicine, organs and drug testing. The potential for tissue regeneration and reconstruction holds the promise of repairing or replacing damaged tissues and organs. This advancement facilitates a more precise examination of the eects of drugs, thereby reducing dependence on animal testing.
Types of Additive Manufacturing (3D Printing):
● Fused Deposition Modeling (FDM): Cost-eective and ideal for prototyping, FDM extrudes thermoplastic material layer by layer.
● Stereolithography (SLA): SLA produces high-resolution prints using liquid resin cured by UV light, perfect for detailed prototypes and intricate designs.
● Selective Laser Sintering (SLS): Utilising a high-powered laser, SLS selectively fuses powdered materials layer by layer, providing versatility in applications.
Advantages of Additive Manufacturing:
● Quick and cost-effective prototyping.
● Production of unique and customized products.
● Construction of complex structures can be done
● Creation of Strong and Lightweight Parts
● Minimization of material waste through layer-by-layer build-up, contributing to sustainability.
● Design modifications can be done without significant cost or time implications.
● Innovative materials such as advanced polymers, metals, ceramics and biocompatible materials.
Challenges and Solutions: Material Limitations:
Challenge: The range of materials available for 3D printing can be limited, especially for certain industrial applications.
Solution: Ongoing research focuses on developing new and improved 3D printing materials, including metals, polymers, ceramics and composites. Innovations in material science expand the range of applications for 3D printing.
Post-Processing Requirements:
Challenge: 3D-printed objects may require post-processing steps, such as sanding, polishing, or painting, to achieve the desired finish.
Solution: Advancements in printing technologies and materials aim to reduce the need for extensive post-processing. Additionally, automated post-processing solutions are being developed to streamline and improve the finishing process.
Print Speed and Production Scale:
Challenge: 3D printing can be time-consuming, especially for large-scale production, limiting its eciency.
Solution: Continuous advancements in printing technology, such as faster printing speeds and larger build volumes, are addressing these challenges. High-speed and large-format 3D printers are becoming more prevalent in the market.
Precision and Surface Quality:
Challenge: Achieving high precision and smooth surface finishes, particularly in certain industries like aerospace, can be challenging.
Solution: Improvements in printer resolution, software algorithms, and post-processing techniques contribute to enhanced precision and surface quality. Additionally, the use of multi-material printing and advanced printing technologies like Digital Light Processing (DLP) can address these issues.
Quality Control and Certification:
Challenge: Ensuring the quality and reliability of 3D-printed parts is crucial, particularly in industries with stringent safety and regulatory standards.
Solution: Certification processes and quality control standards specific to 3D printing are being developed. Non-destructive testing methods, such as CT scanning and ultrasonic testing, are employed to assess the integrity of printed parts.
Education and Skill Gaps:
Challenge: There is a need for a skilled workforce familiar with 3D printing technologies and processes.
Solution: Educational programs and training initiatives are addressing this challenge by providing hands-on experiences with 3D printing technology. Workshops, certifications, and courses are available to bridge the skill gap.
Environmental Impact:
Challenge: Certain 3D printing processes and materials may have environmental implications, such as the use of plastics.
Solution: Startups are exploring more sustainable materials for 3D printing, and eorts are being made to develop recycling solutions for printed objects. Additionally, the use of bio-based and eco-friendly materials is gaining attention.
Future Trends:
● Smart Materials Integration: The future sees the incorporation of smart materials, enabling responsive and adaptive functionalities across healthcare, electronics and more.
● Scale-Up for Mass Production: Anticipate a shift towards large-scale Additive Manufacturing as technology advancements tackle scalability challenges.
● AI-Driven Design Optimization: Artificial Intelligence will be pivotal in optimising design processes, reducing material waste, and pushing the boundaries of complex, lightweight structures.
Conclusion:
In revolutionizing manufacturing with its layer-by-layer approach, 3D printing is a game-changer, opening up unprecedented possibilities for innovation and efficiency. The technology’s impact on reducing costs, enhancing rapid prototyping, and driving innovation marks a transformative era in the manufacturing landscape. As 3D printing continues to evolve, its potential for production efficiency and customization capabilities will certainly shape the future of manufacturing. Embrace the revolution!
Ref:
https://pandorum.com/technology-platform/
https://www.eos.info/en/blog/beginners-guide~b~11957
https://markforged.com/resources/blog/additive-manufacturing
https://www.researchgate.net/publication/257690902_Additive_manufacturing_Technology_applications_and_research_needs
https://amfg.ai/2019/10/08/10-of-the-biggest-challenges-in-scaling-additive-manuf acturing-forproduction-expert-roundup/
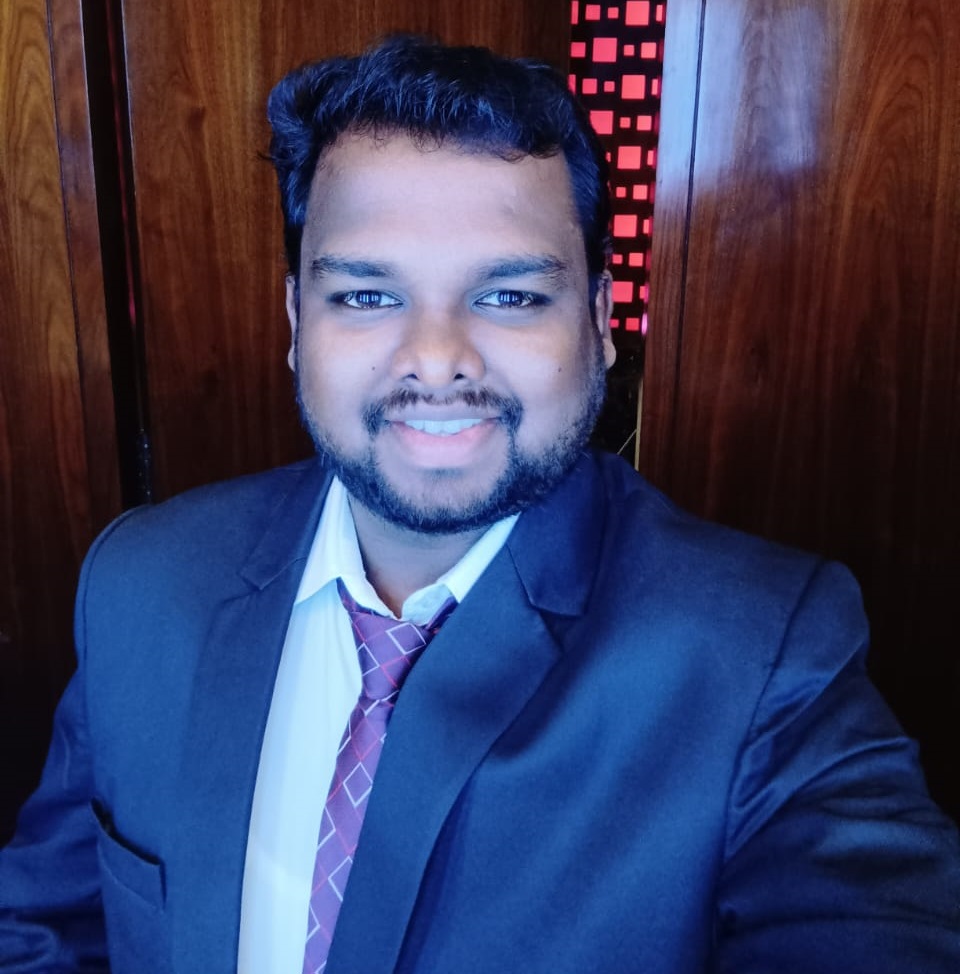
Mechanical Engineer with Proficiency in Innovation and Incubation